EcoHawks Projects
Overall Listing of Past Projects
- 1974 VW Super Beetle Plug-in Hybrid Electric Vehicle
- 1997 GMC JimmE-V
- Active Flow Control
- Altec Analysis of BMS Performance and Behavior Using LiFeMnPO4 Battery Unit
- Altec Battery Chemistry Analysis
- Battery Pack Management
- Black & Veatch Smart Communities
- Biomass Pellet Burner
- Chanje - Last Mile Autonomous Delivery
- Compact Wind Sensor for Electric Bicycle Drag Modeling
- Electric Bike (E-bike) from Used Car Parts
- E-bike Solar Charging Station
- E-bike Design and Manufacturing Year 2
- GEM Swappable Battery Pack
- Greening the KU Field Station
- HEMCO Centrifugal Fan Energy Analysis
- Honda ABS Driving Simulator
- JimmE-V Revisit 2017-18
- Mobile Col-Laboratory
- NREL Wind Turbine Team
- Parallel Hybrid Go-Karts
- Remote Control Cars: Exploration into Batteries, Motors, Fuels, and Hybrid Drivetrains
- Shell Eco-Marathon Urban Concept Team
- Shrouded Wind Turbine and Compressed Air Energy Storage System
- Small Scale Prototype Biomass Drying System for Co-Combustion with Coal
- Small Scale Smart Grid Construction and Analysis
- Small Scale Smart Grid Construction and Analysis Year 2
- Small Scale Smart Grid Construction and Analysis Year 3
- Solar Thermal Greenhouse Initiative
- Soybean Oil to Biodiesel
- Thermal Management of Battery Packs
- Use of Renewable Energy for Heating, Ventilation, and Air Conditioning
- Waste Heat Recovery
- Well-to-Wheel Analysis of Altec Green Fleet
- Westar Prius Battery Pack Re-purpose
- Westar Prius Battery Pack Re-purpose Year 2
Chanje - Last Mile Autonomous Delivery
Chanje is an electric vehicle original equipment manufacturer (OEM) with a specialization in the evolution of the last-mile delivery industry. One of the California-based organization’s grand challenges, and part of their leadership’s ultimate vision, is an entirely autonomous delivery system, to meet the needs of human transportation as well as package delivery. Chanje seeks concept and commercial development assistance from the University of Kansas Mechanical Engineering Capstone Team in addressing this ambitious technical and societal objective. Through application of innovative problem-solving and fresh perspectives, students were tasked with devising a solution that will optimize time and transfer between package and doorstep. Specifically, a robotic arm system subsequently integrated in their delivery vehicle has been designed with versatility and efficiency in mind. Created with the vision of an entirely autonomous delivery, the robotic arm and its respective operational components are capable of package sensing, pick-up and drop-off, and selective placement both inside and outside of the vehicle.
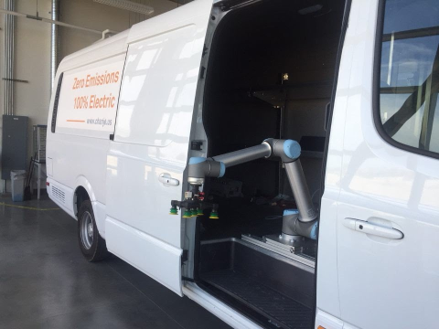
Demonstration of the robotic arm extending from the Chanje delivery truck
Compact Wind Sensor for Electric Bicycle Drag Modeling
Seniors at the University of Kansas developed a thermistor anemometer device to collect wind speed data for Dr. Chris Depcik for the purpose of drag modeling on an electric bicycle. The final product was an additively manufactured (AM) handlebar mounted enclosure comprised of an Arduino microcontroller with data logging capabilities and a wind sensor. The anemometer applies the “hot-wire” technique in which an electric circuit element is heated to a constant temperature and the voltage required to maintain that temperature is measured and recorded. Data acquisition required developing a program for the microcontroller to record the voltage output of the anemometer. Calibration was done by relating the voltage output to wind tunnel wind speed data. The results were conclusive that the data collected were precise in accordance with the wind speeds applied from a closed circuit subsonic wind tunnel. Future studies of this project include a new revision of the wind sensor that includes temperature sensitivity for more accurate modeling, and consequent redesign of the 3D printed enclosure to be compatible with a new internal layout required for the new sensor. Principal conclusions for this project are the creation of a sufficient wind speed acquisition device with a simple platform for modifications and improvements.
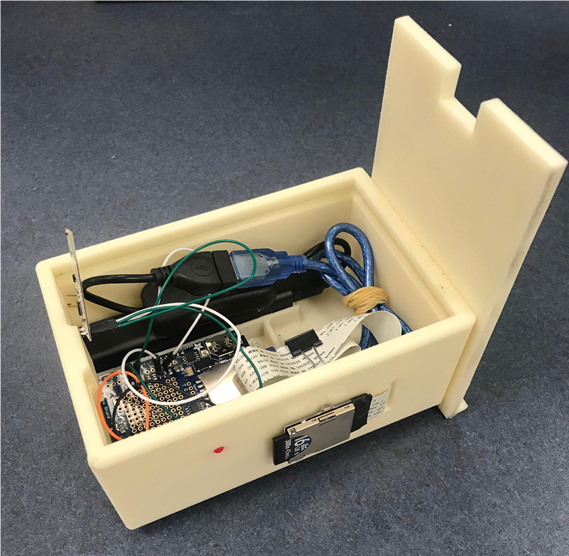
Final product solution highlighting the components within the AM-constructed enclosure
JimmE-V Revisit 2017-18
The cost of gasoline has been rising for decades, and the general population has become interested in the commercial availability of electric and combustion/electric hybrid automobiles and research is needed to understand these vehicles’ capabilities. To do so, the EcoHawks at the University of Kansas have constructed a fully electric car, the JimmE-V, with the purpose of alternative energy and emissions research. The current team’s goal is the restoration of the JimmE-V back to a drivable state including repairs and improvements to several components. This report consists of an overview of the JimmE-V, its components, complications rendering the vehicle immobile, and the solutions to these problems. The main issues involve the battery pack, battery management system, and charging system. Specifically, the battery pack contained several low voltage cells which caused problems when charging. Moreover, an unreliable battery management system was cause for concern due to the fragile cell boards necessary for operation. Additionally, the external charging station would not operate with the battery pack. The team addressed these concerns by reconditioning cells, purchasing a new battery management system, and configuring a wiring diagram for the new system. This project has taught the team problem solving, along with the importance of approaching problems systematically, while searching for innovative solutions. This includes learning project management skills, and the usefulness of thorough record-keeping to keep the team from falling idle. Most importantly, the team has learned about the components and concepts required to build and operate a functioning electric vehicle.
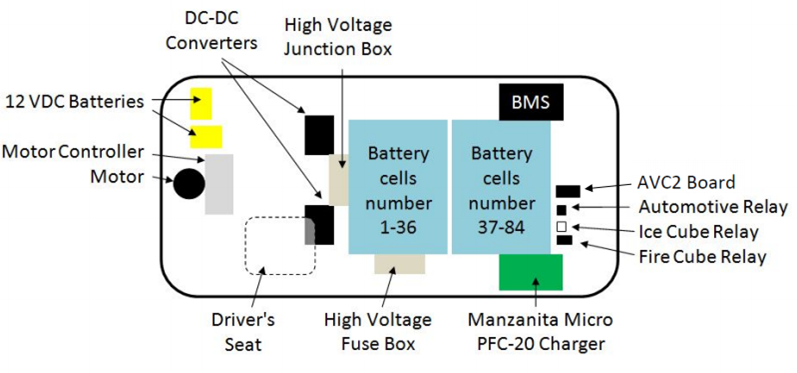
Layout of updated system components in the JimmE-V
Altec Analysis of Battery Management System Performance and Behavior Using LiFeMnPO4 Battery Unit
Altec is a sustainable company in search of ways to reduce fuel consumption and pollution. One of the ways to accomplish this is by implementing battery packs to be used in tandem with utility vehicle engines. To do this efficiently, battery management systems must be used. Battery management systems (BMS) are utilized to monitor performance parameters within a battery pack and balance voltage between cells. Altec’s current BMS is not properly reporting cell temperature, balancing pack cells, and consists of poor hardware robustness. The team was tasked with creating a test apparatus that mimics real world application of the BMS and battery pack to diagnose and solve these issues. After the test apparatus was constructed, it was established that the BMS was misreading the battery pack when cross referenced with raw data from a multimeter and thermometer. Through communication with the project sponsor at Altec, the problem with the setup is believed to be either missing hardware or a BMS on the apparatus meant for another application. The next steps are to debug the test apparatus, develop baseline trends for pack discharge rate, and a cell balancing profile of the BMS to observe Altec’s issues. When these tests commence, complete test procedures will be drawn up to ensure repeatability.
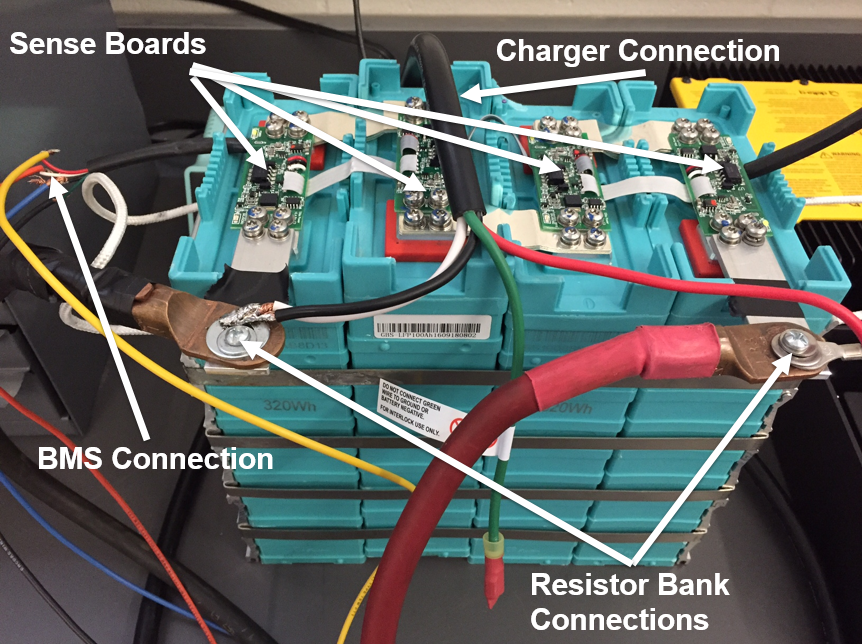
Connection between the Altec batteries and the Orion BMS that controls their overall functioning
Honda ABS Driving Simulator
As the automotive industry transitions to brake-by-wire systems, there is a loss of feedback through the brake pedal that normally occurs when an anti-lock brake system (ABS) is activated. This can have a significant impact on drivers when encountering situations that trigger the ABS. As a result, the goal of this project is to design and implement modifications to the Acura MDX based National Advanced Driving Simulator (NADS) to allow for the testing of driver reactions to ABS notifications and feedback. The ability to utilize three types of feedback to indicate ABS activation will be incorporated into the simulator: pedal vibration along with warning sounds and warning visuals. While the hardware for auditory and visual feedback is already present, the design of an electromechanical control system is essential to the implementation of pedal feedback. Through the design and development of this system, it became clear that achieving the sufficient strength of pedal pulsation and correct timing of the activation of the ABS required significant effort. While the system has yet to produce haptic feedback in the simulator, work continues to complete the implementation and conduct testing.
Movie about the research potential for the driving simulator on campus
Westar Prius Battery Pack Re-purpose Year 2
Climate change concerns are driving incentives to increase the fuel economy of passenger vehicles. Consequently, this has resulted in a growing prevalence of electrified vehicles (EVs) consisting of hybrid, plug-in hybrid, and fully electric vehicles. Unfortunately, EVs are often removed from the road when 70 to 80% of the original energy capacity remains in their battery pack. In order to maintain or increase the value of EV battery packs in an end-of-vehicle life scenario, there are three potential solutions: remanufacturing for re-use, recycling, or repurposing. However, the complexity of handling dissimilar battery chemistries makes both remanufacturing and recycling a significant challenge. Hence, repurposing may prove to be a more viable short-term goal of the industry. In order to explore this potential outcome, a team of undergraduate students studied the continuous cycling effects of used and refurbished Toyota® Prius nickel metal hydride battery packs. A Raspberry Pi 2 Model B microcomputer recorded relevant data, including battery pack voltage, energy input, and energy output. In combination, a Laboratory Virtual Instrument Engineering Workbench (LabVIEWTM) control system used this logged information to regulate charging and discharging of the battery pack. In addition, to enhance the environmental sustainability of the project, this control system acquired solar information from a nearby weather station, subsequently ensuring that the battery pack only recharged during times of peak solar radiation. Analysis of the pack’s energy balance helped to characterize the cycle life of the pack and its potential in repurposing. Others can emulate the methodology employed as a way to instruct students about the potential left in used vehicular battery packs and their possible integration with the electrical grid.
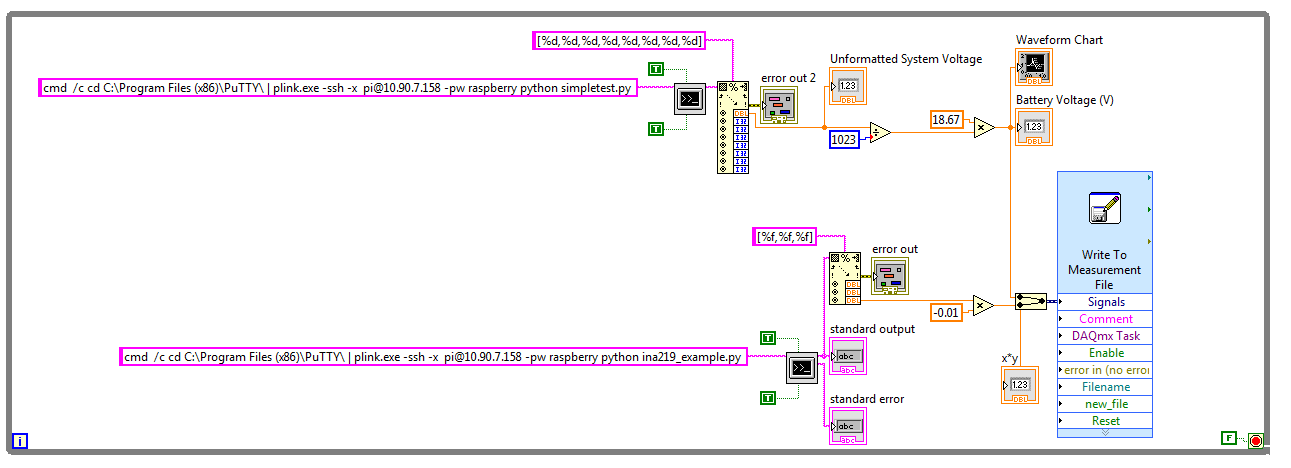
LabVIEW code to enable the secure shell connection to the Raspberry Pi and execute Python scripts for voltage and current value collection. This interprets the data taken by the MCP3008 and INA219, displays it to the Graphical User Interface (GUI), and writes it to a log file.
Smart Grid Year 3
As society moves into the digital age, people and devices are more connected, analytical information is easier to attain, environmental sustainability is a greater concern, and the expectation of instantaneous electricity at the flip of a switch is more prominent than ever. The traditional electric grid composed of central generation with demand-response allocation to consumers has become outdated. To address this, the term “Smart Grid” has been coined to describe a system utilizing powerful software and algorithms, bilateral, interconnected communication networks, and distributed generation resources, to deliver the most reliable and efficient energy to consumers. To better understand the Smart Grid’s ability to influence energy cost, reliability, efficiency, and environmental impact, two years ago, the KU EcoHawks, through sponsorship from Black and Veatch, constructed a scaled version of a Smart Grid. In the subsequent year, the KU EcoHawks have continued their efforts in manipulating the current grid by ways of extensive physical and logic-based changes to enable greater educational benefit, ease of use, data collection, and scenario analysis. Improvements have been made to the model’s circuit design, software interface capabilities, and aesthetic appearance. As a result of these enhancements to the model Smart Grid, the grid can run independently based on constraints related to energy demand, cost, reliability, capacity, efficiency, and environmental impact. Operation of the Smart Grid model based on manipulated and varying constraint-controlled scenarios will be analyzed to study Smart Grid components’ performance, energy distribution efficiency, and energy economics.
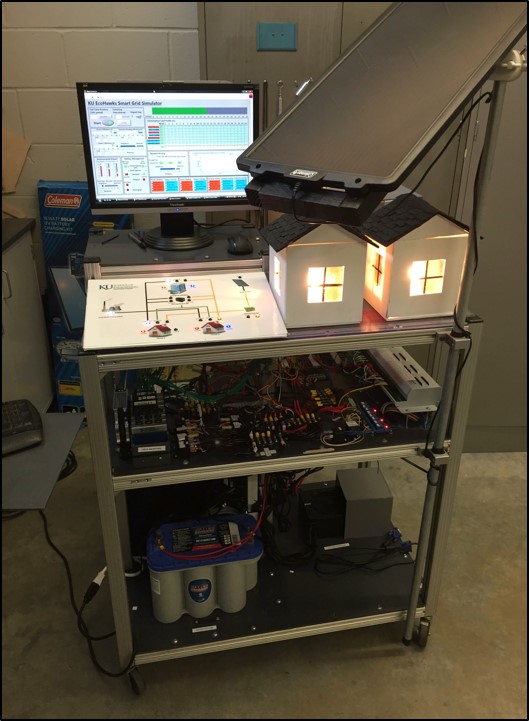
Complete smart grid setup: The buildings and control rests on the top shelf, the middle shelf has the wiring and relays, and the bottom shelf includes the power generation and storage devices
Soybean Oil to Biodiesel
Presently, the University of Kansas (KU) has a state of the art facility where researchers generate biodiesel from soybean oil. However, these investigators need both a production unit that can produce oil from soybeans and a small batch biodiesel refinery to encompass the entirety of their Feedstock to Tailpipe Initiative (FTI). Due to this, effort was dedicated to determining the most viable method for extracting oil from soybeans along with designing how to create small batches of biodiesel from this oil. Past efforts researching methods for extracting oil from soybeans revealed that a mechanical press is a more effective and sustainable extraction method when compared to solvent extraction. With respect to the various types of mechanical extraction techniques, hot pressing is the most effective for soybeans. Due to various complexities, high prices, and past attempts at engineering a working mechanical press, the best option unearthed was to purchase a $360, professionally manufactured press allowing for the creation of approximately six gallons of soybean oil per day. The primary focus then shifted to the construction of a small-scale refinery. This chosen route consists of a steady-state, constant volume, adiabatic closed loop system that will be powered by a 12 VDC pump and capable of handling roughly six gallons of inputted oil for a price of around $1,500. The unit will apply the necessary heat for transformation from oil to biodiesel and glycerin by way of a heat exchanger. Upon completion, KU will have the ability to test and enhance all aspects of their FTI process.
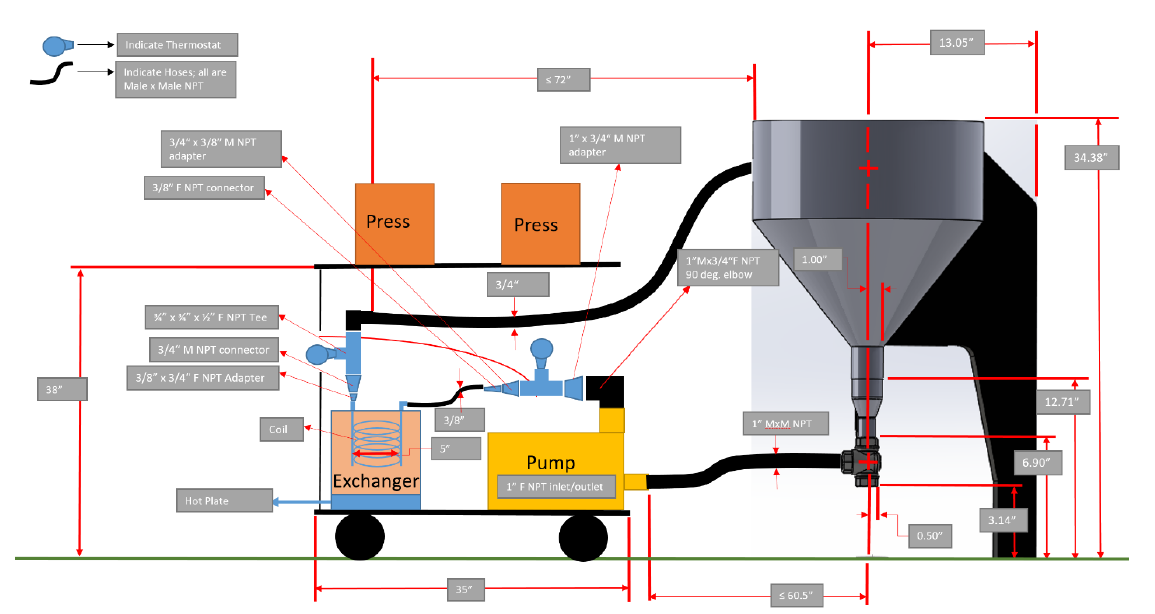
Final design for the overall biodiesel production process including the soybean oil presses
Westar Prius Battery Pack Re-purpose
The 2007 Toyota Prius is equipped with a 202 VDC battery pack that retains a significant amount of its initial capacity after the vehicle reaches its vehicle end-of-life. As these and other electric vehicles are taken off the road, researchers have investigated the efficiency and potential applications of these second life batteries. Re-purposing and recycling these battery packs will create an alternative energy source, while offering a solution to an imminent waste problem. To test the second life efficiency of a used Prius battery pack, work will be done to configure the battery pack for powering two radiant heaters in the winter and a large fan in the summer in the Hill Center’s mechanical pod on East Campus at the University of Kansas. The subsequent research was broken up into two components: energy in and energy out. Voltage measurements verified that the battery provided by Westar Energy is still valuable for this application. The battery, and its various electrical components, will be reconfigured to produce the AC voltage required by the heater. At the same time, the battery pack and component-housing unit is being designed to comply with specific customer requirements. Continuous analysis of the battery’s capacity, and its charge to discharge relationship, is required to justify the practicality of real world applications. Circuit analysis indicates that the battery pack needs to be configured in parallel branches of 14.4 VDC, with an inverter supplying 120 VAC to the radiant heaters.
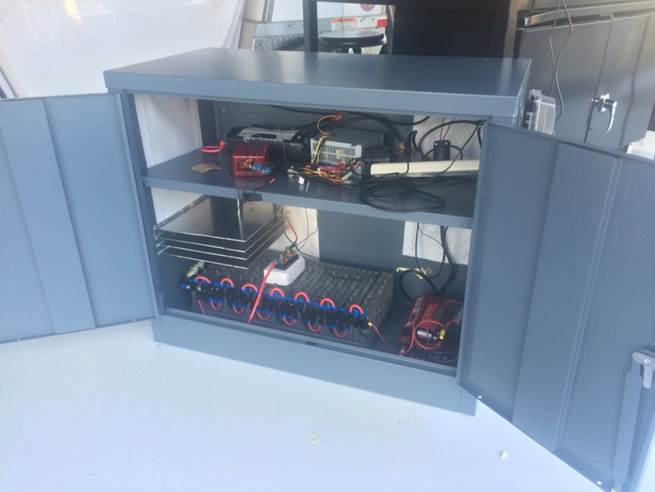
Housing system for battery pack with used Prius battery pack on the bottom shelf
Altec Battery Chemistry Analysis
Lithium ion batteries are a growing technology that has many applicable uses. Hybrid car technology uses a typical combustion engine while also utilizing lithium ion battery power for different car processes. Altec equips utility trucks for many different working applications and would like to introduce this technology to their trucks to become more environmentally friendly and efficient. Altec has asked for testing and data on different chemistries of lithium batteries. Using EcoHawks’ sustainability metrics (ethics, environment, economics, and energy) a ranking system will be developed to better educate Altec on their battery selection process. Hybrid technology has yet to be effectively applied on a large scale to utility trucks. Due to the recent influx of new battery technology, there is a lack of battery chemistry understanding and how to apply it effectively in specific applications. Many different battery chemistries were researched to formulate a narrow list of potential lithium batteries for testing. A Vencon Universal Battery Analyzer was used for the testing and data collection of the purchased batteries. Given the battery usage profile from Altec, the batteries were cycled until 50% initial capacity and were compiled into a rankings matrix. Temperature data was also tracked and incorporated into these rankings for further understanding and applicability. Through the research and analysis of several battery chemistries, Altec will be able to implement more efficient and environmentally friendly utility trucks into larger scale production. In the future, Altec hopes to test larger batteries in an environmentally controlled atmosphere to gain a better understanding of these batteries and to better apply different battery types to their utility trucks.
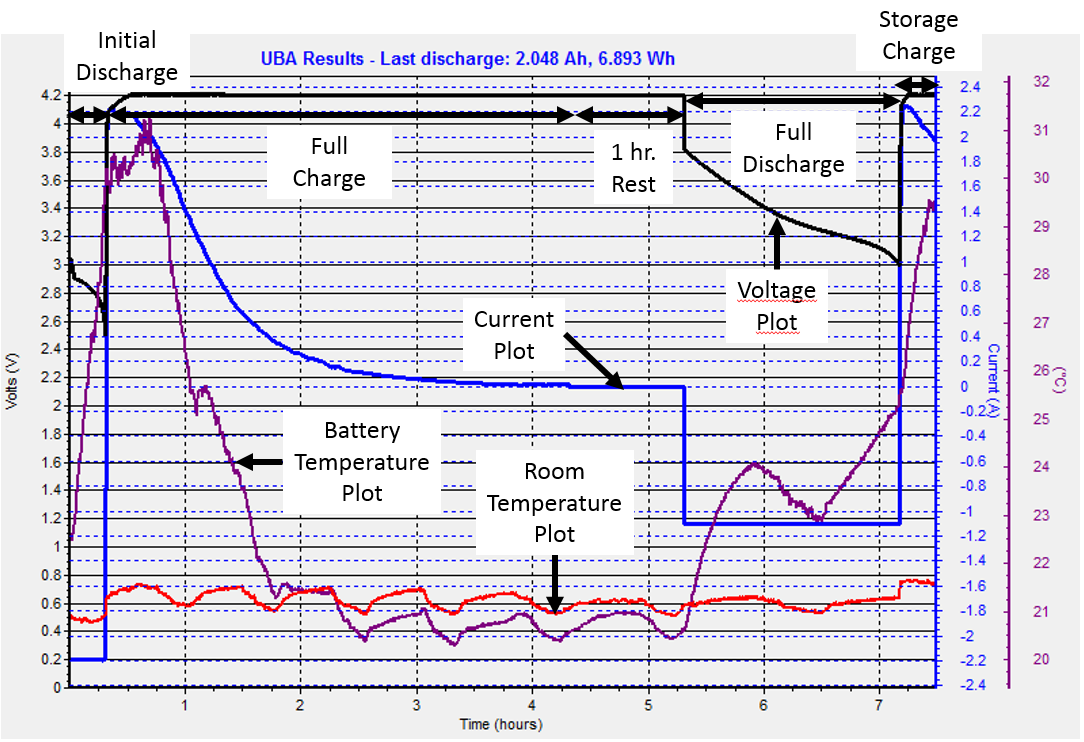
A sample of a graphical representation of battery test results from the Vencon machine over a charging and discharging profile input by the user
E-bike Solar Charging Station
The future of energy is one of the biggest concerns the world faces today. As greenhouse gas emissions continue to pollute the environment at an alarming rate, the need for alternative energy is increasing. One promising solution to this ever-growing issue is solar powered energy to power various transportation. The idea of utilizing electric vehicles has been growing. However, there is still the issue of where this energy comes from. In order to address this issue the Electric Bike Solar Charging Station will utilize clean and sustainable energy to charge the electric bike designed by the EcoHawks last year. Making the switch to clean energy is an extremely difficult task; not only do electric vehicles need to be manufactured and marketed, charging stations need to be readily available for the consumer to use. With electric transportation in its early stages, more research and development is needed in order to make this a viable alternative to current gasoline powered vehicles. Creating a clean and sustainable way to charge these vehicles would be a great step in the development of this promising technology. Our design will include adjustable photovoltaic solar panels that charge a battery bank that will then fully charge an electric bike overnight. Research will be conducted on important design aspects including but not limited to: solar panel angle, type of battery bank, charging controller, and solar irradiation. Based on the success of this project, the solar charging station could potentially be implemented throughout campuses across the country. By utilizing solar powered charging stations, the emissions from transportation to and from these campuses will be significantly reduced.
The EcoHawks e-bikes within the solar charging station
Active Flow Control
Active flow control is the process of expending energy in order to reduce drag and increase lift on aircraft lifting surfaces in order to improve fuel economy. The goal is to increase efficiency through the use of fluidic oscillators, which function by forcing air through fixed flow channels to create a sweeping jet that minimizes boundary layer separation. While active flow control works well in a laboratory setting, there has been little advancement in actual flight test hardware, primarily due to cost and scaling issues. In particular, different geometries would be required for high and low speed fluid velocities. This project focuses on designing a flight-ready system of fluidic oscillators that would work in both low speed and high speed applications. The fluidic oscillators would then be installed on an unmanned aerial vehicle (UAV), and tested both in a laboratory setting and at the North Lawrence Airport. With the growing emergence of UAVs for use in personal, commercial, and military applications, the necessity for this research is increasing rapidly. Any increase in the efficiency of a UAV can dramatically increase its range and flight time, which are critical for the completion of many missions. These improvements will hopefully result in an increase in fuel efficiency of 1-2%.
Looking down the wing at the fluidic oscillators embedded within the wing of an UAV
E-bike Year 2
In today’s society, those who don’t take advantage of public transportation services typically will drive their vehicles to school, work, or another area of interest, even though the distance may not be very great. Riding a bike or walking tends to be avoided due to the amount of physical exertion that it requires. This creates a concern when the emissions generated by the use of motor vehicles account for a large percentage of greenhouse gasses released into the atmosphere. This, along with the ever increasing gas prices creates a need for an alternative means of travel over shorter commutes. An electric bicycle is one such solution. Electric bikes don’t demand the physical exertion which walking or riding a traditional bike would require, and also utilizes an energy source that has a less significant impact on the environment. Because the energy required for fabrication of custom bike parts also creates a negative impact on the environment, the bike will be made from as many recycled car parts and scrap material as possible. A focus on the aesthetics of the bike will be maintained so that a sleek and attractive design will be achieved. For this to be accomplished, an initial design will be made with the idea of using all brand-new bike parts in mind. It will then be modified accordingly once the recycled materials are obtained. Once the bike is fabricated, testing will be performed on battery life, average speeds, the bikes ability to traverse various routes including those with many hills, and other aspects of its functionality. The final result will be a bike that is appealing in both its appearance and effectiveness to those making short ranged commutes, and has the potential to greatly reduce carbon emissions in urban areas should its use become widespread.
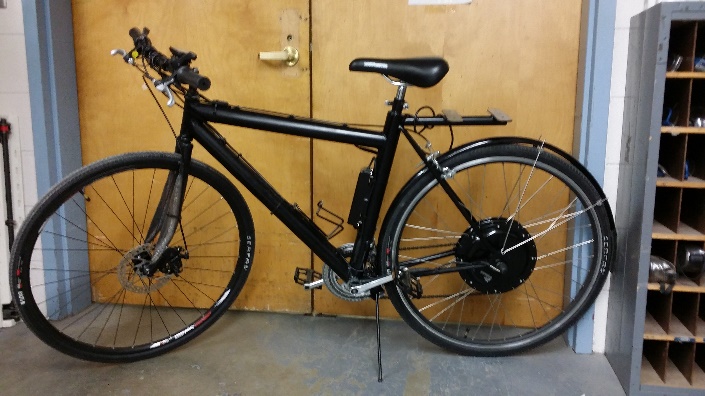
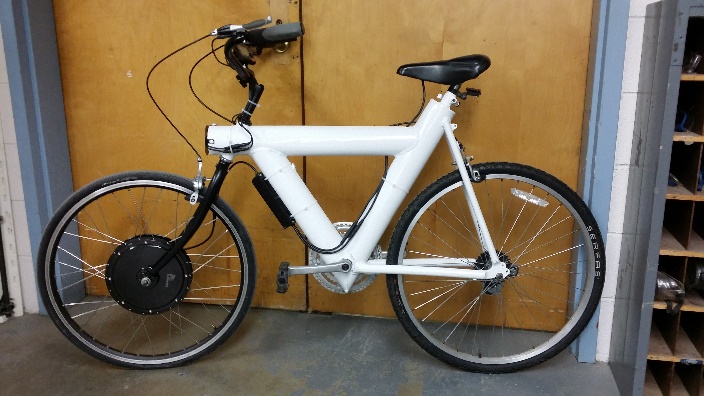
(Top) Second year's e-bike after powder coating; (Bottom) First year's e-bike after a few modifications and powder coating.
Thermal Management of Battery Packs
With the escalating goal of decreasing the consumption of fossil fuels, the creation of an effective thermal management system to support and encourage the use of fuel cells and battery packs in electrically powered vehicles is to be explored. The desired result is to create a thermal management system for a fuel cell or battery pack to prevent it from overheating, its by-products from freezing, and ideally maximize its efficiency. The thermal management system would consist of phase change materials (PCMs), made from wax, surrounding the energy source (or substitute heat source during testing). The thermal management system will make use of the latent heat of the PCM to store released thermal energy during operation and supply energy back to the energy source when dormant. Configurations of heat pipes within the various PCM designs would also be used to increase the heat flow to the PCM, as wax PCM has very low thermal conductivity. The solid-liquid two-phase heat transfer mechanism of the PCM would be investigated through experimental measurements of temperature profiles of the energy source and the PCM during operation and when idle. The effectiveness of the thermal management system would be examined at both hot and freezing environmental conditions. The thermal management system would ideally maintain the energy source in its ideal range of operating temperatures for operation and when idle, regardless of the weather condition. If these results are achieved, fuel cells and electric batteries can be more effectively incorporated into everyday vehicles operating under all weather conditions, vastly improving the realization of more electric-powered options on the market.
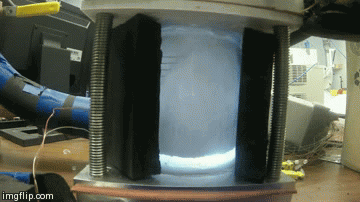
Testing of the PCM during an active heating event
Waste Heat Recovery
Internal combustion engines lose approximately one third of the fuel’s energy through the exhaust as heat. With the EPA ever increasing the exhaust emission standards, this wasted energy could be recovered and used to increase efficiency throughout the system while reducing emissions and saving on fuel costs. To answer this problem, our team was directed to employ the Organic Rankine Cycle to recover the waste heat generated by the combustion to be used as electrical energy. This cycle has been implemented in power plants on a large scale but has not been widely used in internal combustion engines even though it works well with the temperature range of a car’s exhaust. Wasted energy through the exhaust has been recovered in the past by turbochargers. An alternative to implementing an Organic Rankine Cycle would be to utilize a turbocharger to operate the generator directly. The problem the turbocharger is that it can only use instantaneous energy from the system and is dependent on RPMs while being less efficient. However, the organic Rankine cycle continually feeds from the system without being parasitic or dependent on RPMs. This project aims to achieve a potential fuel economy increase of up to 5% using water as the working fluid. This is compared to a typical efficiency in a Rankine cycle of 10-20%. Further miniaturization of our system could be applied to passenger vehicles to further optimize their efficiency. The generated electrical power could be used to power accessory equipment such as the air conditioner, lights, coolant pump allowing for downsizing the energy the alternator has to produce.
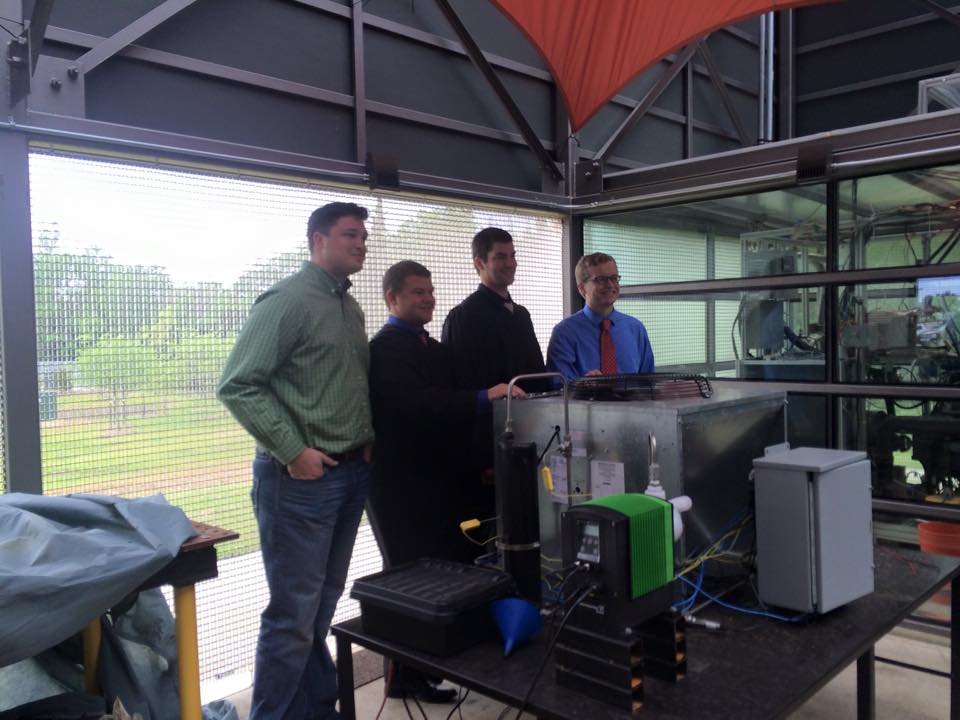
The team posing in front of their Organic Rankine System setup
Battery Pack Management
With the increasing usage of electric vehicles around the globe, battery pack management systems are now becoming more important than ever, to ensure an energy efficient and cost effective means of transportation. Our team is proposing to design and build a 36 VDC battery pack that will be used to power the EcoHawks Electric Bike. The initial goal is to improve the existing battery pack system in place on the first generation E-bike. The current battery pack is permanently secured to the bike; however, we propose a design for removable batteries, allowing them to be exchanged and thus eliminating downtime for charging. We plan to add a number of auxiliary features to the battery pack that will provide the rider with more useful information, such as remaining battery life, and researchers with useful data, such as a “running data collection” system, that can continuously monitor various parameters. For our approach, we will first conduct a review of current battery pack technology applicable to our technical requirements, including a review of current commercially available e-bikes. We will then select a battery and configuration for our pack that will optimize power, capacity, weight, and geometry. We will collaborate with the E-bike team to determine pack placement, connections, and design the exchange-ability function. Finally, we will determine which auxiliary functions we wish for the pack to perform, including those that will enhance the riding experience and those that will assist research and experimentation. The vision for our end product will be a “smart” and exchangeable battery pack, which the rider can take with them when not on the bike, and replace when dead. The pack will provide the rider with pertinent information, and will also monitor properties such as temperature, voltage, amperage, and capacity so that data analysis can be performed on the E-bike. These improvements relate to the EcoHawks mission by improving the energy efficiency of the battery pack and (for the E-bike as a whole) providing an energy-moderate form of transportation.
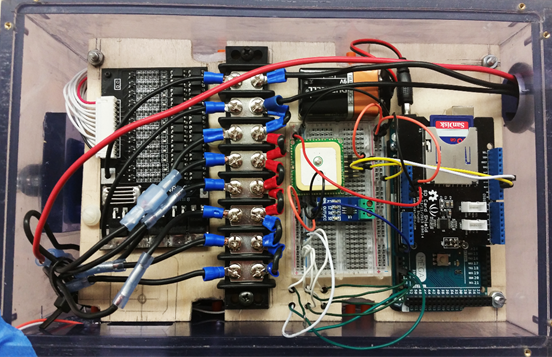
Completed and working battery pack illustrating the wiring and internal components
Smart Grid Year 2
Communities of the 21st century face hardships that are different than any other era. With the increase of people, limits in resources and need for environment conservation, public a demand for energy efficiency is steadily growing. Advances in computing power and software capabilities have made smart grid technology a viable solution to this problem. The smart grid utilizes control systems and sensors to transmit data in two-way communication and distribute power as needed. The grid monitors itself and reacts in real time to changes in demand/supply of power. The grid can feed off of the main centralized source, but also takes advantage of local, renewable distributed generation. The local grid can also sell power back into the central line and/or use energy storage devices during times where production exceeds demand. In the case of a power surge or outage, the grid can function independently of the main line. EcoHawks will build a smart grid model that incorporates three separate model structures with varying power demands and supply capabilities. The model will include a power source to represent the centralized grid, an energy storage device, and a solar panel. A compact Rio will operate to control the smart grid using programming written by our team. This will be modular and will serve, at the very least, as a robust base model from which scale-up ability is possible. This model will provide data on cost and efficiency to contribute to an optimal grid system for a small residential area. From this research we should understand how we use what's currently built to figure out how smart grids should be incorporated into the existing system. This should allow future optimization and improvement in energy efficiency in the true grid.
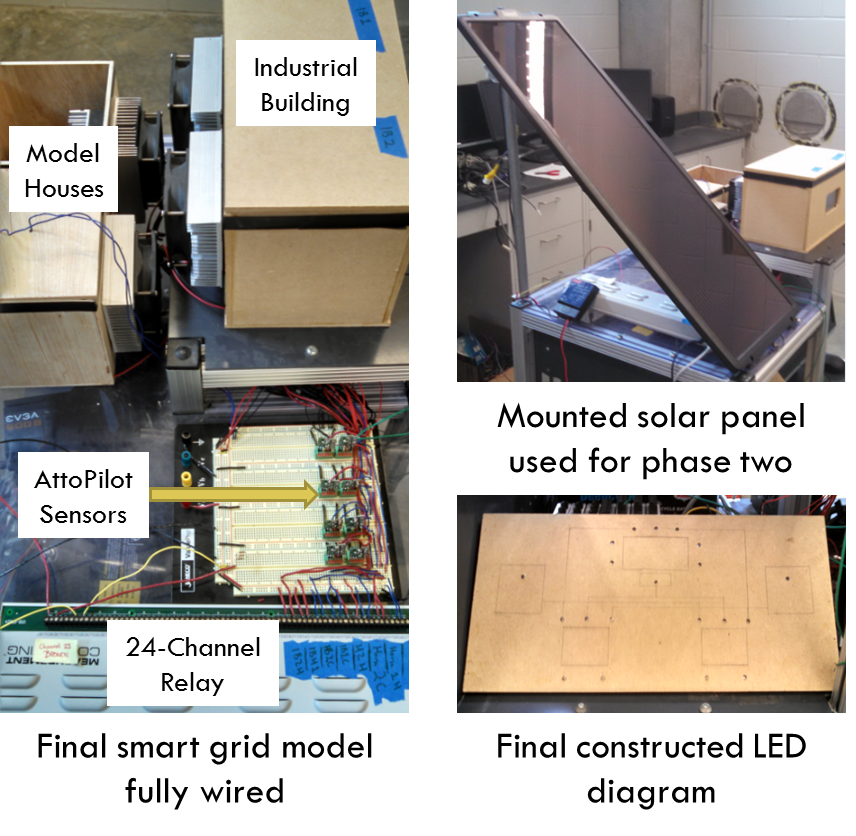
Final smart grid illustrating the analogs employed for control and renewable energy
HEMCO Centrifugal Fan Energy Analysis
Note: this project is a blended effort with Dr. Dougherty's class. The purpose of this project is to design and use an apparatus for testing several centrifugal exhaust blowers provided by HEMCO. This apparatus will be able to test each size of fan provided (4”, 6”, 8”, 10”, 12”) at a duct velocity between 1200 and 1500 ft/min. It will be able to hold 10 ft or 40 lbs of pipe while occupying an area smaller than 5’x3’x10’. The procedure(s) for using this testing apparatus will also be developed by the team and will be able to be repeated by a third-party within 1% root-mean-square error. It will be able to be set up by a single technician in 10 minutes and the duration of the test will be no more than 10 minutes. The output data of these tests will include for each fan: Fan flow curve (volumetric flow rate vs. static pressure change,) energy usage as a function of back pressure, duct velocity as a function of back pressure, and face velocity as a function of back pressure. In addition, a computational fluid dynamics model will be developed for as many fans as time permits, and recommended modifications in fan enclosure design will be given.
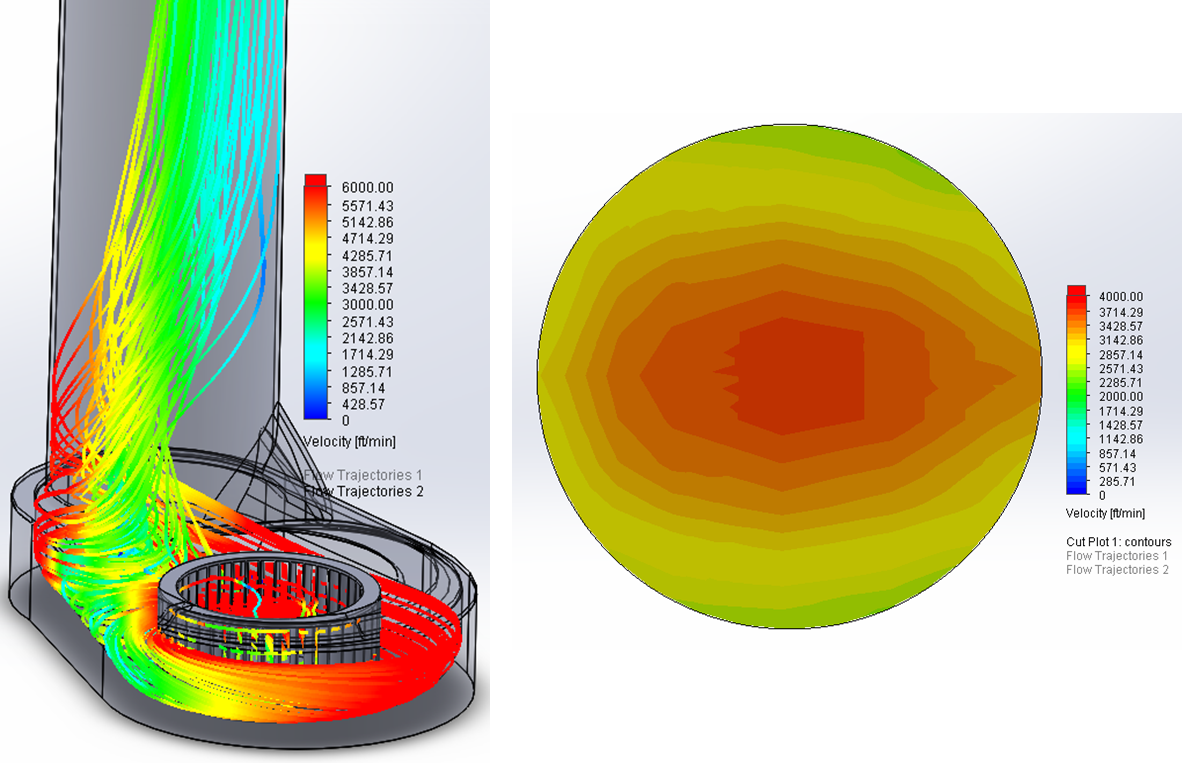
Computational Fluid Dynamics simulation example of the velocity streamlines for a HEMCO centrifugal exhaust blower
Shell Eco-Marathon Urban Concept Team
Students compete in the Shell Eco-Marathon competition within the Urban Concept division using the previous year's vehicle (updating and fixing it) while fabricating a new vehicle for the following year. This methodology allows for eight months of testing and learning to improve next year's design instead of rushing to finish and not testing at all. This program teaches real-world applications of minimizing cost while obtaining maximum output (reducing $/MPG is the overall goal).
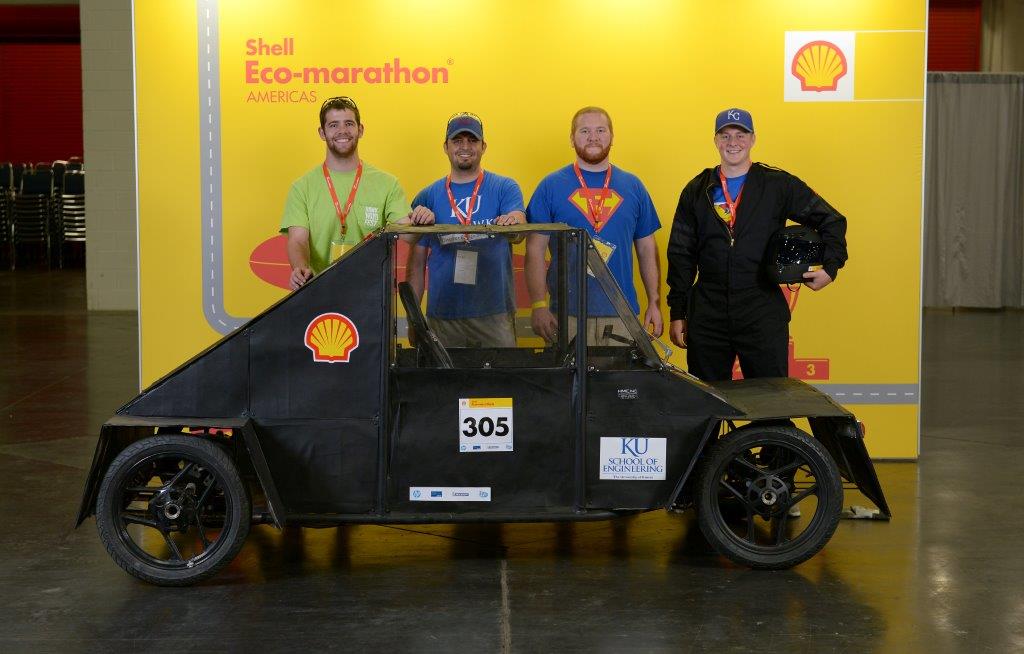
The 2013-14 Urban Concept team with Uncle Kevin at competition; best run of the team was 94 miles per gallon
Electric Bike from Used Car Parts
This project will be designing and building an electric bike using as many parts from old cars as possible. Parts that cannot be salvaged from a car will be taken from old bikes and more important parts will be bought or donated new. An electric motor from E-BikeKit will be added to help aid the ride for the electric component of the project. The bike will need to be able to travel from Eaton to the Hill Center and back on one charge of the battery, using the motor to more easily ride up inclines. The objective of the project is to promote more energy efficient and sustainable methods of transportation.
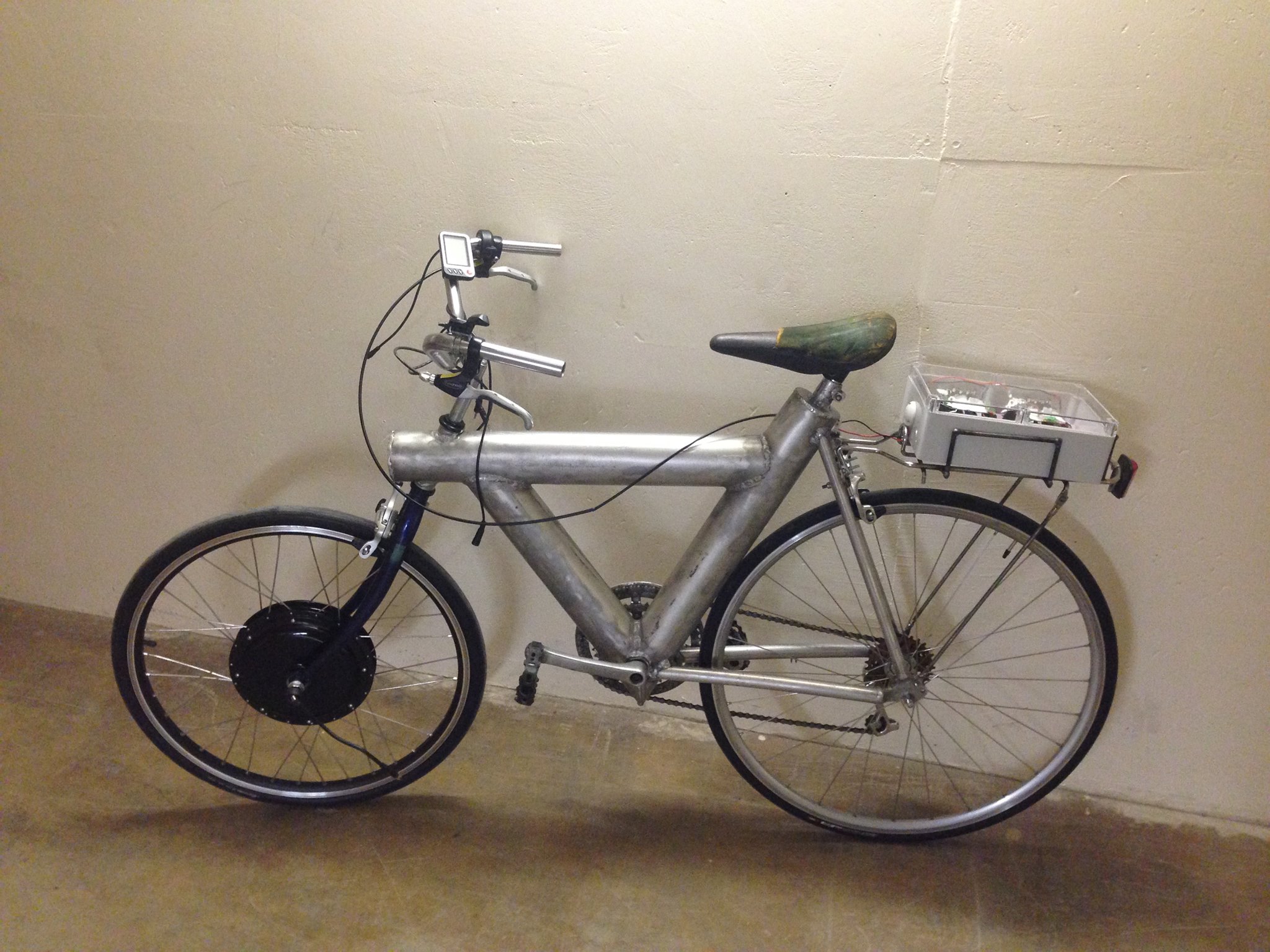
Finalized e-bike resting against the wall highlighting the robust frame constructed from a truck running board
Mobile Col-Laboratory
A number of faculty are working together on the renovation of an Airstream trailer to function as a mobile col-laboratory for civic engagement. A team of EcoHawks are working to design and implement a low energy, audio-visual system with Internet capabilities that can be used by the university to travel through KS (and elsewhere) to: develop methods and spaces to foster one-on-one collaborative, interactive planning and design to imagine publicly-shared spaces that foster positive community life, promote engagement through art and culture, and foster healthy lifestyles in communities surrounding Lawrence, Kansas and beyond. The EcoHawks work will also include the sizing and selection of a biodiesel generator to power the audio-visual system. The team also will investigate the potential installation of flexible solar panels on the roof to remove some of the load from the generator.
Movie about the MoCoLab designed for researchers to establish connections to local communities
GEM Swappable Battery Pack
As the flaws of fossil fuels become more ever-apparent, alternatives are being developed to fill the gap. There has been a large initiative to produce electric vehicles (EVs) to replace internal combustion engines (ICEs) that currently populate the roads. However, EVs are less capable of traveling greater distances and providing as much power as ICE vehicles; but when used at slow speeds and for short commutes, EVs are operationally cheaper and a more effective means of transportation. The University of Kansas (KU) Center for Sustainability currently is in possession of a Global Electric Motorcar (GEM) that will be primarily used to collect recycling from the KU campus. This vehicle was in disrepair and required replacement of the lead acid batteries. The Center for Sustainability has donated the vehicle to the KU EcoHawks so that they may recondition the vehicle. This team has upgraded the batteries in the GEM with lithium ion chemistry which will decrease vehicle weight and increase the available range. In addition, a battery drawer has been added to the vehicle providing the ability to rapidly exchange battery packs and facilitate a longer working day. The construction efforts have provided the GEM with an easy-to-use, swappable battery pack that is much lighter and better accessible than the standard GEM battery pack. The renovation is expected to increase battery and usage efficiency, ameliorating the effectiveness of the vehicle in transporting personnel and recycling while improving the campus environmental impact and outlook.
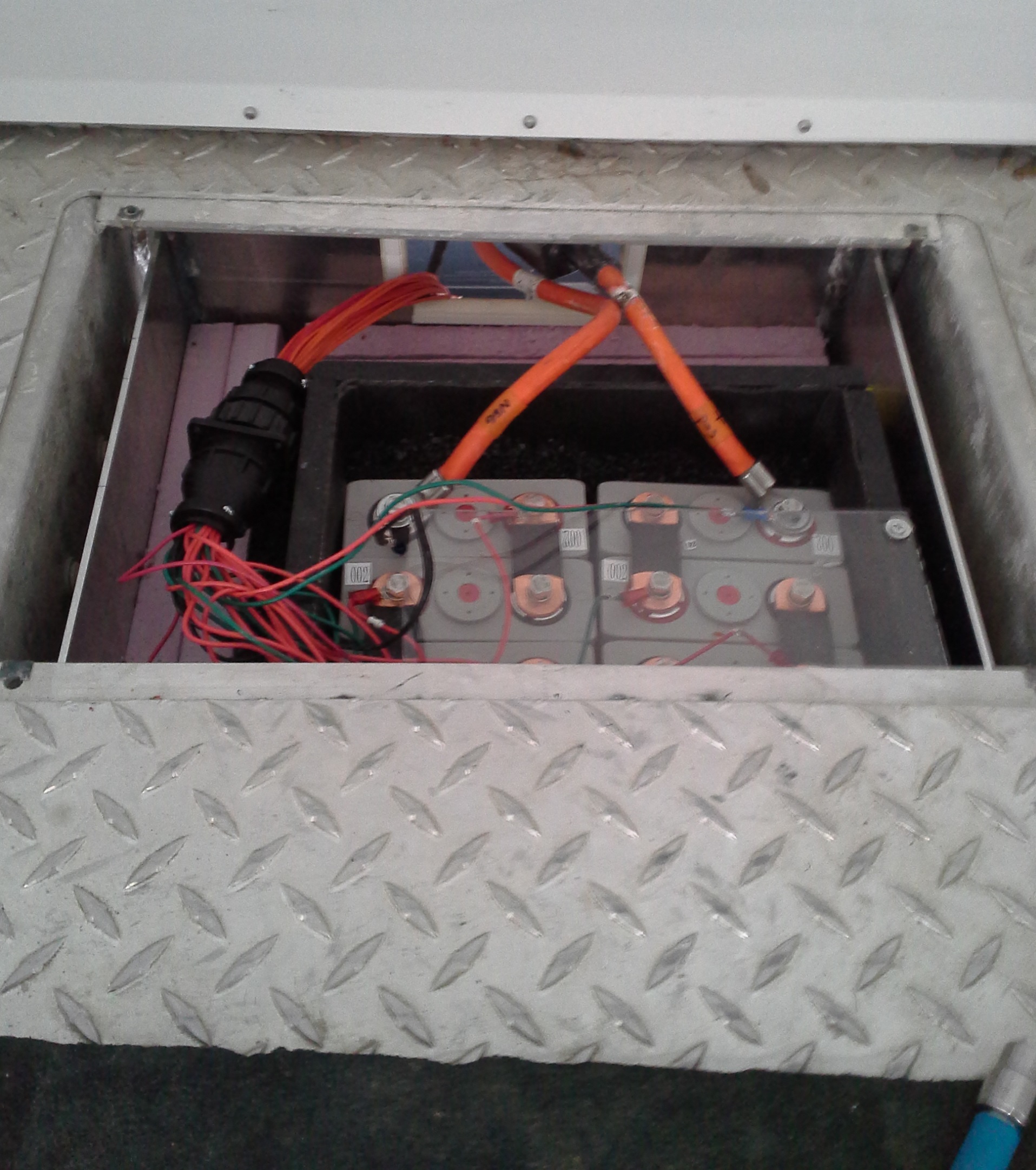
Connection location of the replaceable battery pack to the electric motor
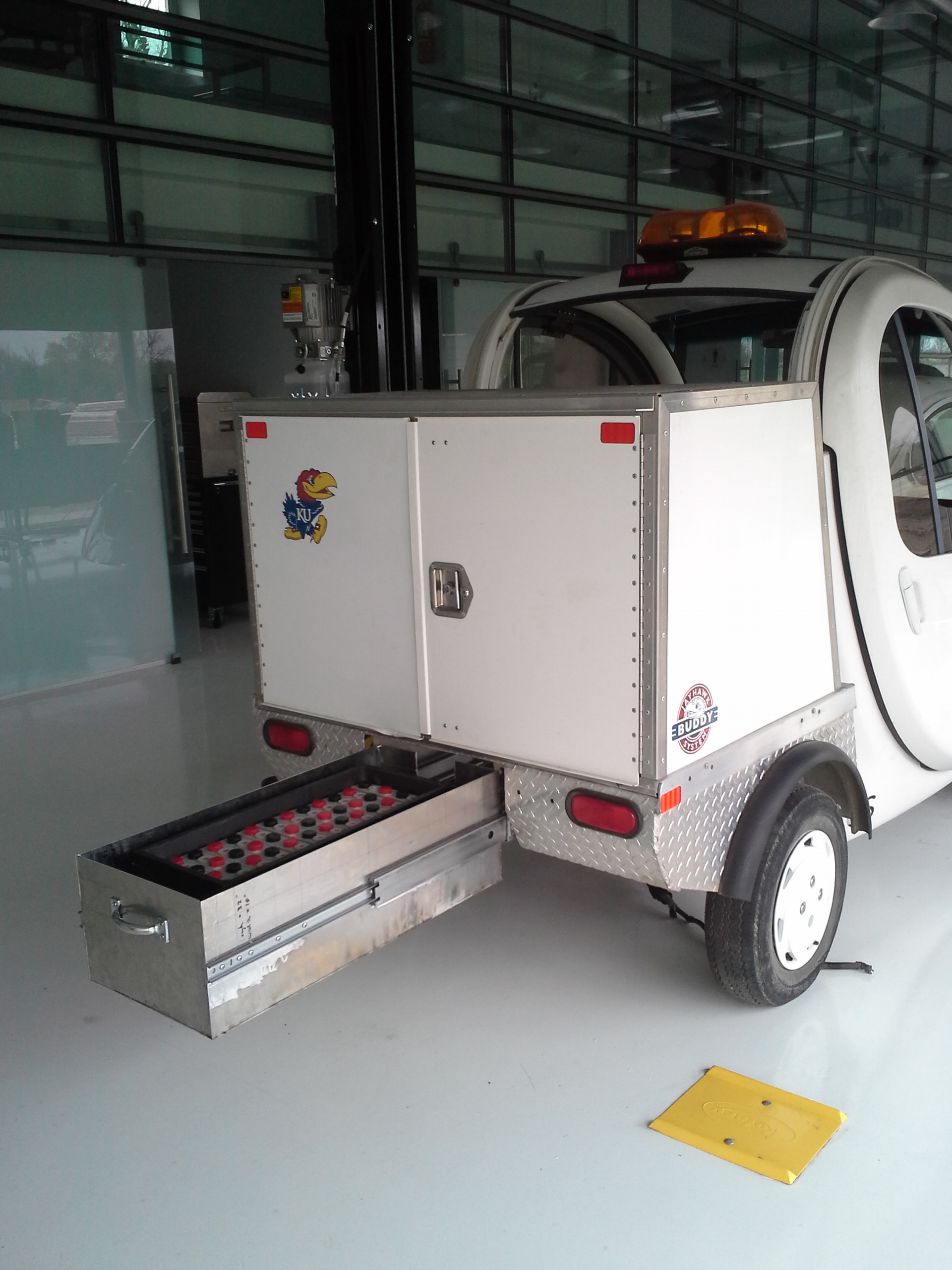
Demonstrating the battery tray extended from the vehicle
Biomass Pellet Burner
This team designed a solid biomass pellet burner in which a fixed bed is used to test the combustion of solid biomass fuels under various oxygen concentrations. The goal is to test emissions of said fuels, especially dried algae, to see if they are a viable "green" alternative to fossil fuels or suited for co-combustion with traditional fuels, such as coal, in order to reduce emissions. The students performed various flow rate and mechanical/thermal stress calculations while implementing a National Instruments data acquisition system.
Second test of the glow plug ignition system in order to initiate combustion
NREL Wind Turbine Team
The U.S. Department of Energy (DOE) Collegiate Wind Competition challenges undergraduate students from multiple disciplines to design and construct a lightweight wind turbine. A group of KU EcoHawks teamed up with business and aerospace engineering students to design and construct a quality turbine that performed according to a customized, market data-derived business plan. The team traveled to Las Vegas in May for the competition, where the turbine was tested for parameters such as cut in wind speed, rated power control, and emergency shut down, while the accompanying business plan was evaluated. Overall, the team finished in second place while placing first in Testing & Design and the Business Plan sections.
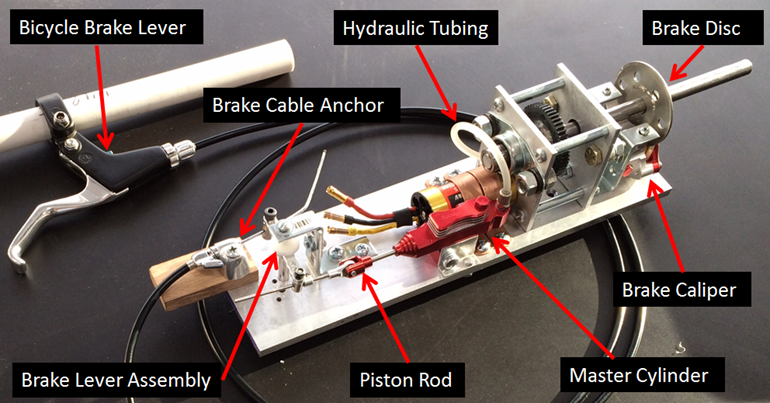
Disc brake system of the competition turbine also illustrating the fabricated gearbox
Successful shutdown of the turbine at competition
One version of the electronics test bed that was employed by the team
Black & Veatch Smart Communities
An industrially sponsored project where a team of students will build a series of scale "homes" and control them as a function of ambient temperatures, energy availability, and cost as well as an input consumer demand while including a renewable source of energy. The team will develop a control system for use in manipulating the different sources of electricity to a smart community in order to reduce grid power dependency. This investigates the green component of the smart communities of the future by integrating solar panels and battery storage into the smart community infrastructure.
Scale homes built with connections to the grid and lead-acid battery (proxy for grid storage) including relays and data collection system
Well-to-Wheel Analysis of Altec Green Fleet
Altec's Green Fleet consists of different Aerial truck models that have been modified to run their aerial systems off on-board batteries and diminish the dependence of an idling engine at the job site. The purpose of the project is to develop an automated data acquisition system to obtain fuel consumption and power data from this fleet. These numbers are used to obtain emission information from the ANL GREET software. With these figures, a more detailed and more marketable cost saving calculator can be produced to help Altec in the sale of their more efficient fleet.
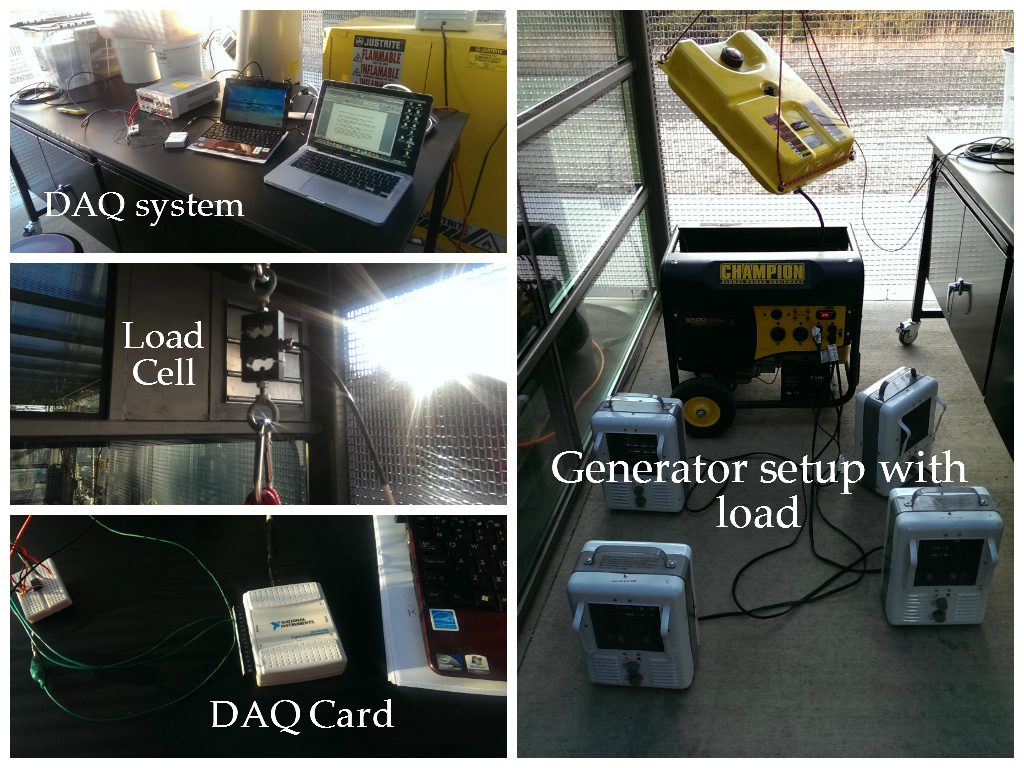
Higher resolution testing system measuring the fuel consumption from the generator (analog to Altec engine during operation)
1997 GMC JimmE-V
The JimmE-V is the EcoHawks’ second full scale electric vehicle conversion. From 2010 to 2013, students applied engineering design principles to develop an innovative alternative energy vehicle using current automotive technology. The JimmE-V is a purely electric vehicle with a 35 kW-hr battery pack. The battery pack is composed of 104 lithium iron phosphate (LiFePO4) batteries. The battery pack supplies 330 VDC to the Azure Dynamics motor controller, which inverts the DC power to AC power before sending it to the 56 kW three-phase AC motor. The motor is directly coupled to the differential using a custom drive shaft. The motor controller is controlled over a Controller Area Network (CAN), and communicates with an electronic control module (ECM) to interface with the accelerator pedal. The ECM also controls regenerative braking commands, and displays the accelerator pedal position and motor torque for the driver. The JimmE-V also has a Battery Management System (BMS) that communicates over the CAN network to ensure that the battery cells are evenly charged and discharged. The JimmE-V also uses an electric air-conditioning compressor, electrical heating, and an electric power steering pump to provide typical amenities found in standard automobiles. Along with a fully customizable display, the JimmE-V has thin-film solar panels on the roof to allow the 12 VDC auxiliary battery to charge. In order reduce external noise, sound deadening material was applied to the wheel wells and interior. The vehicle will allow further research on electric vehicle modeling, battery aging, and Well-to-Wheel analysis.
JimmE-V preparing for the first test drive
Patrick Collins driving the JimmE-V for the first time
1974 VW Super Beetle Plug-in Hybrid Electric Vehicle
The low emission and high fuel economy standards set by regulatory agencies are causing an increase in the number of electrified (hybrid, plug-in hybrid, and battery electric) vehicles reaching the marketplace. In order for students to obtain a better understanding of the architecture of these vehicles, a 1974 Volkswagen Super Beetle was converted into a plug-in series hybrid electric vehicle (PHEV) with a generator that runs on used canola oil biodiesel. Moreover, this vehicle is connected to a solar photovoltaic charging station for a comparison of recharging on or off the electrical grid. By performing a materials life cycle energy and emissions analysis using GREET2.7 (from Argonne National Laboratory) on parts reused in the conversion to an PHEV, over 2,400 kg of CO2 emissions and 31.79 GJ of energy use was avoided due to the processes required in re-manufacturing or recycling the old parts and manufacturing new components. In a Well-to-Wheel study, when utilizing rapeseed oil biodiesel and the 2010 US average electricity consumption mix, the emissions range from 150 to 199 g of CO2 per km depending on how the vehicle was operated. However, the implementation of a 1.1 kW solar photovoltaic fueling station facilitates an improvement to 146 g of CO2 per km. Overall, energy use was found to be as low as 0.318 kWh/mi with a maximum fuel economy reached of 106.1 mpge and maximum speed of 78 mph.
The PHEV Super Beetle sitting outside its home at the Hill Engineering Research and Development Center
Austin Hausmann takes the PHEV Super Beetle for a ride
Small Scale Prototype Biomass Drying System for Co-Combustion with Coal
Thermoelectric power plants burn thousands of tons of non-renewable resources every day to heat water and create steam, which drives turbines that generate electricity. This causes a significant drain on local resources by diverting water for irrigation and residential usage into the production of energy. Moreover, the use of fossil reserves releases significant amounts of greenhouse and hazardous gases into the atmosphere. As electricity consumption continues to grow and populations rise, there is a need to find other avenues of energy production while conserving water resources. Co-combusting biomass with coal is one potential route that promotes renewable energy while reducing emissions from thermoelectric power plants. In order to move in this direction, there is a need for a low-energy and low-cost system capable of drying materials to a combustion appropriate level in order to replace a significant fraction of the fossil fuel used. Biomass drying is an ancient process often involving the preservation of foods using passive means, which is economically efficient but slow and impractical for large-scale fuel production. This effort instead implements an active drying system for poplar wood using theorized waste heat from the power plant and potentially solar energy. The use of small-scale prototypes demonstrate the principles of the system at a significantly reduced cost while allowing for calculation of mass and energy balances in the analysis of drying time, Coefficient of Performance, and the economics of the process. Experimental tests illustrate the need to distribute air and heat evenly among the biomass for consistent drying. Furthermore, the rotation of biomass is critical in order to address the footprint of the system when placing next to an existing thermoelectric power plant. The final design provides a first step towards the refinement and development of a system capable of efficiently returning an amount of biomass large enough to replace non-renewable resources.
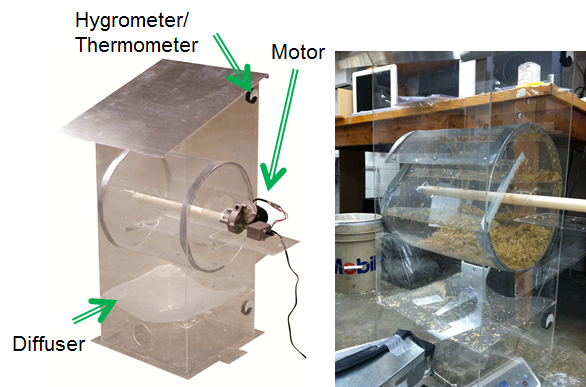
On left, second prototype constructed prior to testing with the photo on the right demonstrating the testing with the motor removed
Small Scale Smart Grid Construction and Analysis
Designed with a singular purpose, the electrical grid currently used in the United States (US) is formed around the purpose to “keep the lights on”. It is a centrally planned and controlled system with relatively little flexibility to fluctuations in energy demand. As the nation and the economy becomes increasingly digital, energy demand is growing rapidly. While the automotive industry is presently dependent on petroleum sources, the growing presence of Plug-in Hybrid Electric (PHEV) and Battery Electric Vehicles (BEV) will begin to act as a stress upon the electrical grid by drawing resources during times of peak energy demand.Of importance, taking a sustainable approach towards these vehicles can act to lower greenhouse-gas emissions, improve urban air quality, save consumers money, bolster power-grid reliability and reduce oil imports. Toward this objective, the EcoHawks applied for and received an EPA grant (P3: People, Prosperity and the Planet) to build a stand-alone model of a scale Smart Grid.
Overview of the small scale Smart Grid developed by the EcoHawks
Members of the EcoHawks show off the Smart Grid
Nicholas Surface describes the Smart Grid
Shrouded Wind Turbine and Compressed Air Energy Storage System
As the push for renewable energy sources continues, one significant drawback over fossil fuels is that they are not reliable. Wind is not guaranteed at all times, and the sun does not always shine. Moreover, the demand for electricity is variable due to daily and seasonal swings in power draw from the grid. Conventional power plants can increase or reduce production to meet seasonal demand, but usually cannot meet daily fluctuations. Therefore, power plants must maintain a relatively high level of electrical generation capacity throughout the day even if the current demand is low. A prime place to focus upon electrical demand fluctuations and the unreliability of the renewable sources is at the location of the changes in demand. Homes often sit vacant throughout the day and draw little power from the grid. When residents return in the late afternoon and evening, a sudden increase in demand occurs. To address this increase in demand at a local level, a small-scale proof-of-concept shrouded wind turbine (SWT) and compressed air energy storage (CAES) system was designed, built, and tested. The concept is that a small SWT charges the CAES system and when the residents return, the energy stored within the CAES system is released lessening the demand on the main electrical grid. The SWT was investigated due to the theorized increase in efficiency that the shroud provides by accelerating the air beyond ambient velocity at the location of the turbine blades. The CAES system consisted of a three-stage compressor that filled a high-pressure scuba tank. This air was then released in a controlled manner in order to operate an air motor coupled to an alternator that generated electricity. Testing of the SWT found that the prototype was too small to power the compressors for the CAES; however, the concept of the SWT was shown to hold true. Experiments using the CAES system demonstrated significant losses, but it did generate electricity. The small-scale prototype did reveal that the idea of focusing on the source of the power fluctuations is a viable option. As a result, by using many small power production and storage devices, the overall daily swings in demand for electricity can be corrected to levels that current power plants can meet.
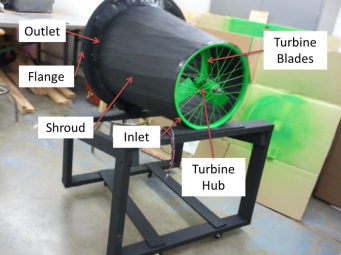
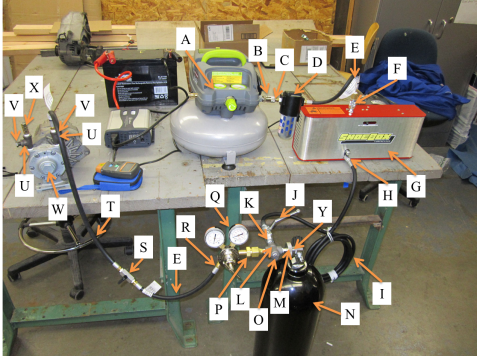
Of the left is the completed SWT illustrating the shroud, flange, and turbine, and on the right is the CAES system components after assembly
Greening the KU Field Station
The goal was to design and build a stationary photovoltaic system that will collect enough energy to sustain the Berm Building at the KU Field Station located North of Lawrence, KS. The team produced the best design within the economic limitations of the project while investigating the possibilities and benefits of building a solar tracking photovoltaic system through prototyping and experimentation. Furthermore, a thermodynamic model of the Berm Building was generated in order to calculate the current and future demands of energy. This included testing solar cells and comparing the outcomes to pyranometer readings in order to calculate the respective efficiency of the cells. These metrics, among others, were used to select and size the solar cells for the photovoltaic system. The sixteen solar panels installed reduce the energy usage at the Berm Building by 30%, generate 682 kWh/month, and save the University of Kansas approximately $74/month. Finally, the team’s research provides support to future students tasked with continuing to green the KU Field Station.
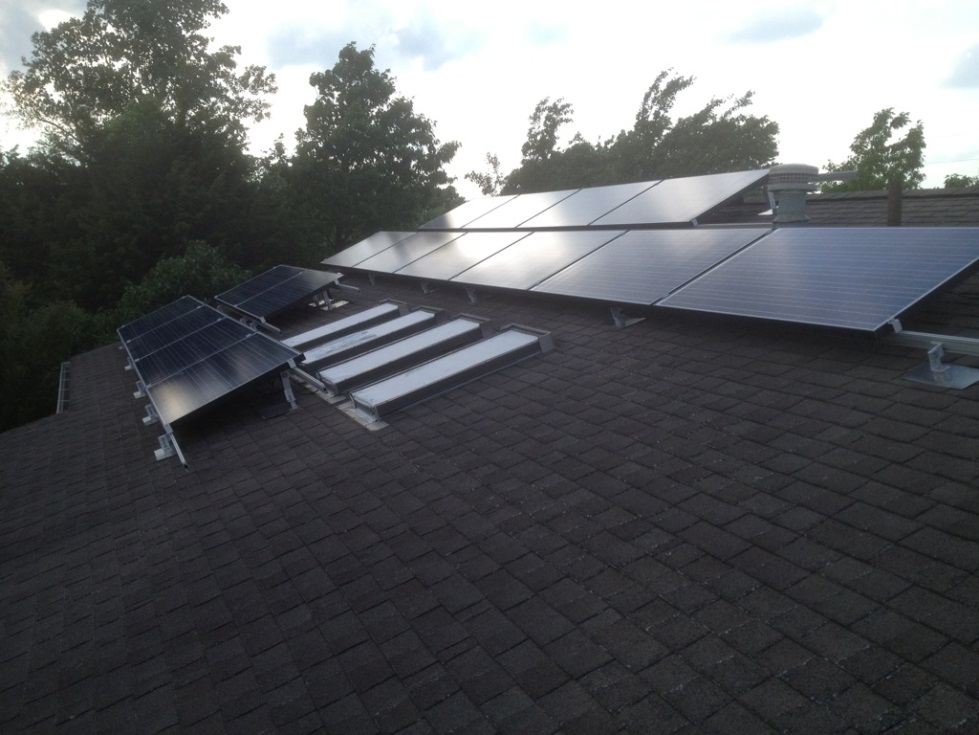
The installed solar panels on the Berm Building at the University of Kansas Field Station
Remote Control Cars: Exploration into Batteries, Motors, Fuels, and Hybrid Drivetrains
The Engineering Education and Centers wing of the National Science Foundation has undertaken the task of re-structuring engineering education. The objective is to make it more effective, quality conscious, flexible, simpler, and less expensive. Past EcoHawks have designed and constructed Remote Control (RC) cars in order to integrate the design and build process into the ME curriculum. RC cars are an effective teaching tool as it helps focus the analysis of these ideas while increasing pertinence to current social topics. It builds better engineers by creating a curriculum-wide methodology that merges theory with practice generating better quality designs. The flexibility of the program is evident in the different designs using varying levels of technology, such as parallel hybridization, brushed and brushless DC motors, fuel cells, hydrogen, biofuels, and lithium batteries. Small-scale building is inherently simpler as parts are easier to manufacturer and mistakes can be readily eliminated and components redone. Finally, at around $1000 per 1/8th scale car, the costs of the program are significantly reduced making the project feasible for all universities, community colleges, and even high schools of various sizes.
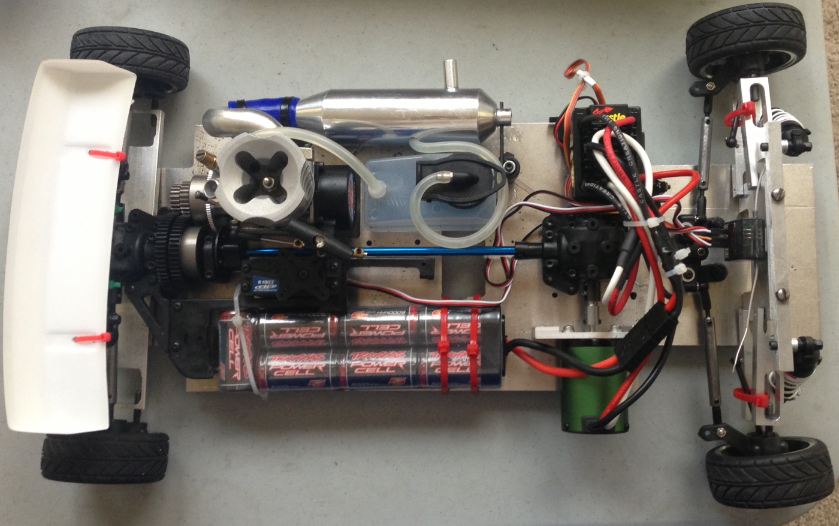
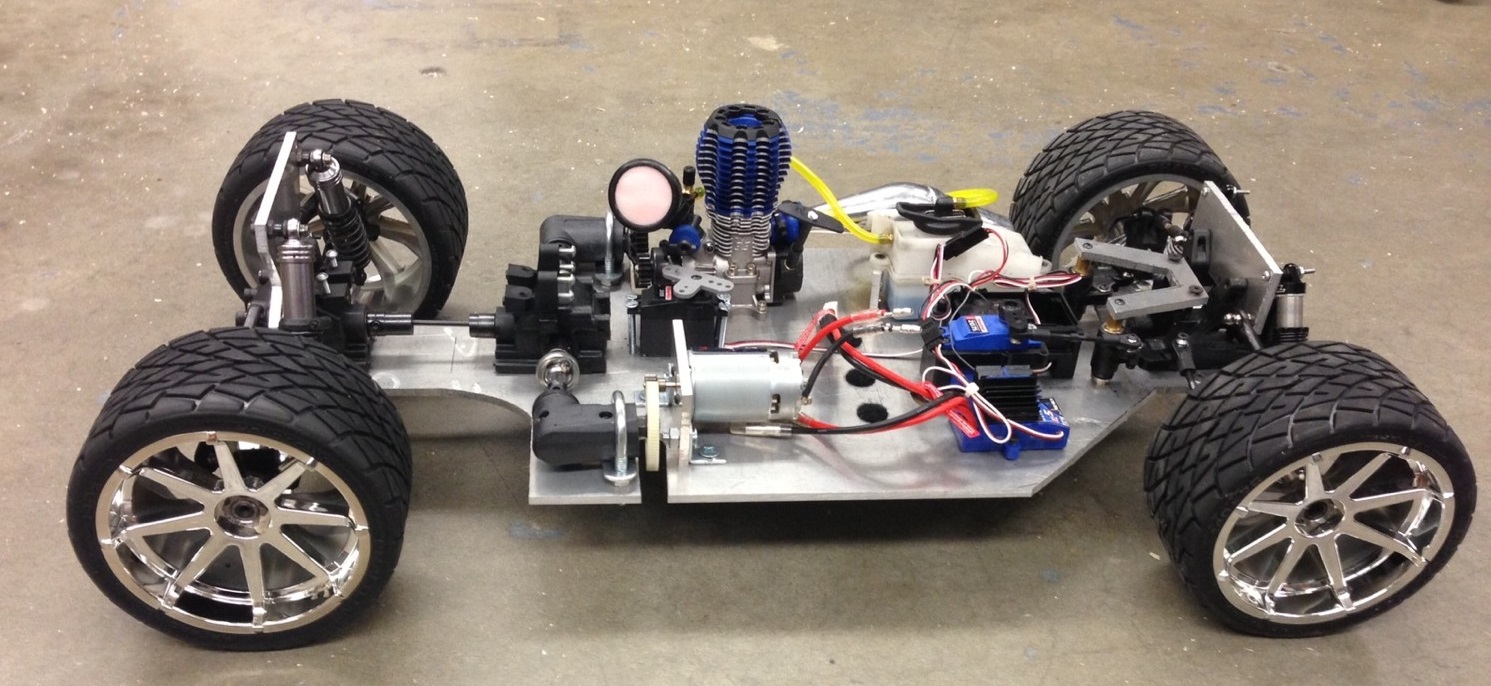
The two latest versions of parallel hybrid Remote Control cars developed by EcoHawk students
Parallel Hybrid Go-Karts
Two teams of EcoHawks built parallel hybrid go-karts as our start into the construction of sustainable automobiles (seen more recently in the Urban Concept project). This vehicle design is straightforward and inexpensive enough to be understood, assembled, and modified by high school students. Ultimately, the goal of the project was to promote sustainable engineering for future generations with one of the vehicles donated to a local high school. The project will hopefully expose high school students to a tangible project that combines engineering with energy and applies to the future of automotive technology.
Having fun driving around the parallel hybrid go-karts
Solar Thermal Greenhouse Initiative
The objective of this project was to use solar energy in order to provide heat for the Biology Department’s greenhouses. This system was to use six 4 ft. x 10 ft. flat plate solar collectors to gather and focus solar energy in order to heat a glycerin-water mixture flowing through a pipe. This pipe would have traversed the greenhouses, and connected to a thermal storage unit. This unit was to be insulated, which would prevent heat loss while storing energy until needed by the greenhouse. A second circuit would carry hot fluid from the storage unit into the greenhouse, heating the interior environment by means of convection. This would have ideally keptthe greenhouse within a temperature range of 65°F to 75°F. In order to maintain this temperature range, multiple factors, such as environmental conditions and geometry of the system, were considered. Unfortunately, the costs and required location of the solar thermal collected were deemed prohibitive. However, many lessons were learned in the areas of converting active and passive solar energy to heat.
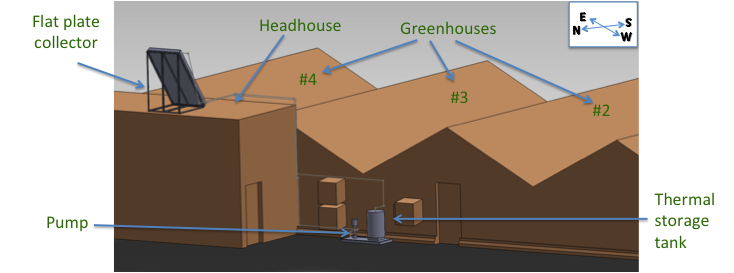
SolidWorks image of the proposed system layout
Use of Renewable Energy for Heating, Ventilation, and Air Conditioning
An EcoHawks team designed a renewable energy powered heating, ventilation, and air conditioning (HVAC) system for the previous University of Kansas EcoHawks barn. In a climate where temperatures can become extreme in both the summer and winter seasons, HVAC is important for thermal comfort and healthy working conditions for the students. Their efforts covered the energy sources powering the system, its main components, how final design decisions were made amongst the team members, and how the design relates to sustainability. The team's final design included a heat pump with the main source of energy being a solar parabolic trough. The solar panels on the roof of the barn were utilized to power the electrical components of thesystem. Heat energy coming from the solar trough was absorbed by the working fluid, a 70% glycerin-30% water mixture, and stored using an insulated tank. From the tank, hot fluid will flow through radiators within the barn and heat the air within. A prototype was developed, experimentation yielded the necessary sizing of system components, and the heat collection side of the system was installed in the barn.
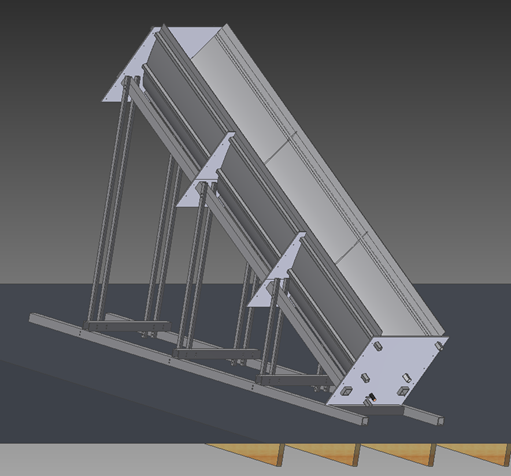
Computer Aided Drafting image of the parabolic trough and frame mounted on the roof at 45°